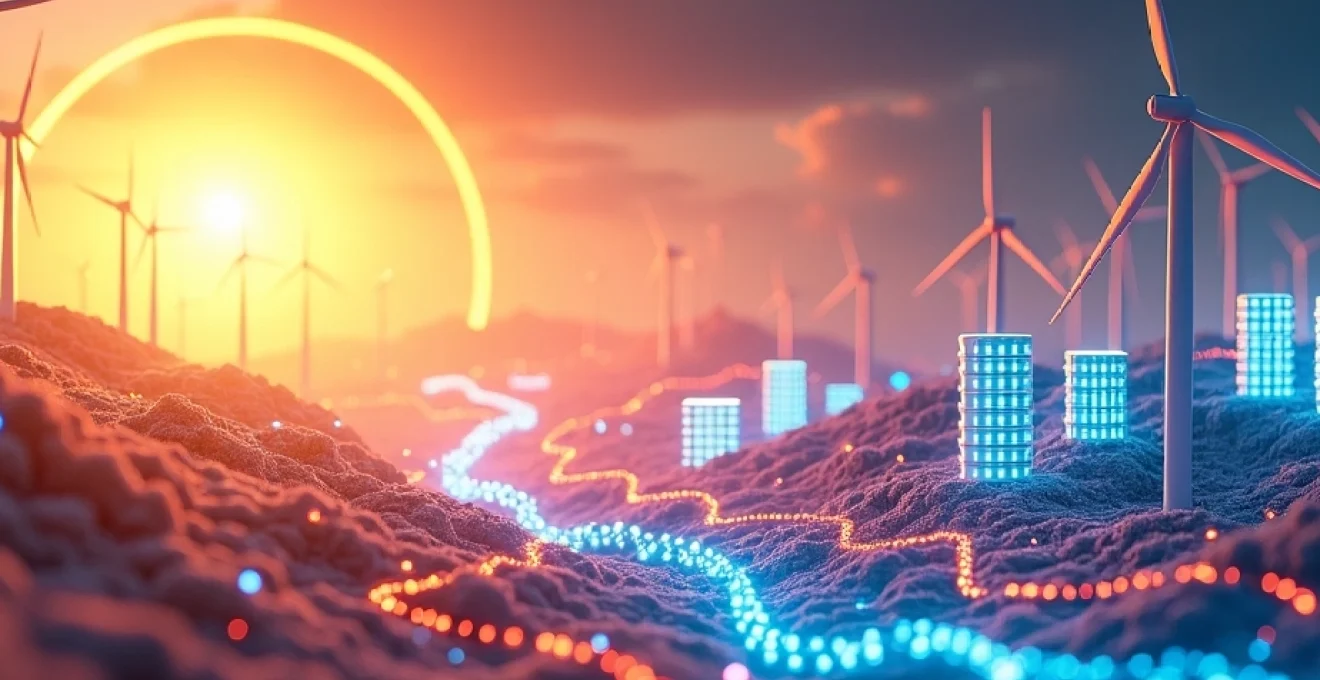
The global shift towards sustainable energy solutions has placed renewable energy at the forefront of technological innovation. As the demand for clean power grows, so does the need for more efficient energy conversion systems. These systems play a crucial role in harnessing and utilizing renewable energy sources, transforming them into usable electricity for homes, businesses, and industries. Recent advancements in energy conversion technologies are revolutionizing the renewable energy sector, paving the way for a greener and more sustainable future.
Thermodynamic principles in energy conversion systems
At the heart of every energy conversion system lie the fundamental principles of thermodynamics. These laws govern the transformation of energy from one form to another and set the theoretical limits for efficiency. Understanding and optimizing thermodynamic processes is crucial for engineers and scientists working to improve renewable energy technologies.
The Second Law of Thermodynamics, in particular, plays a pivotal role in energy conversion efficiency. It states that no thermal system can be 100% efficient, as some energy will always be lost as heat during the conversion process. This principle drives researchers to develop innovative methods to minimize these losses and push the boundaries of efficiency closer to their theoretical limits.
One approach to enhancing thermodynamic efficiency is through the use of combined cycle systems. These systems capture waste heat from one process and use it to power another, effectively increasing the overall efficiency of the energy conversion. For example, in solar thermal power plants, excess heat that would otherwise be lost can be used to generate additional electricity through steam turbines.
Thermodynamic optimization is not just about minimizing losses; it's about creating synergies between different energy conversion processes to maximize overall system efficiency.
Advanced materials and nanotechnology are also being leveraged to improve heat transfer and reduce thermal losses in energy conversion systems. For instance, nanostructured surfaces can enhance heat exchange efficiency, leading to better performance in solar thermal collectors and heat engines.
Advanced power electronics for renewable energy integration
Power electronics serve as the critical link between renewable energy sources and the electrical grid. These sophisticated devices are responsible for converting, controlling, and conditioning the power generated by renewable sources to make it compatible with grid requirements. Recent advancements in power electronics have significantly improved the efficiency and reliability of renewable energy systems.
Wide-bandgap semiconductors in solar inverters
One of the most promising developments in power electronics is the adoption of wide-bandgap semiconductors, such as silicon carbide (SiC) and gallium nitride (GaN). These materials offer superior performance compared to traditional silicon-based semiconductors, enabling higher switching frequencies, lower losses, and improved thermal management in solar inverters.
Solar inverters equipped with SiC or GaN components can operate at higher temperatures and voltages, leading to increased efficiency and power density. This translates to more compact and cost-effective solar power systems, capable of converting a higher percentage of solar energy into usable electricity.
Multi-level converters for wind turbine applications
In the realm of wind energy, multi-level converters are gaining traction for their ability to handle high-power applications more efficiently. These converters use multiple voltage levels to create a smoother output waveform, reducing harmonic distortion and improving power quality.
For large-scale wind turbines, multi-level converters offer several advantages:
- Improved efficiency at partial load conditions
- Reduced filter requirements, leading to smaller and lighter designs
- Better fault tolerance and reliability
- Enhanced grid support capabilities
These benefits contribute to the overall performance and grid integration of wind energy systems, making them more competitive and reliable sources of renewable power.
Smart Grid-Tie inverters with adaptive control algorithms
The integration of renewable energy into the grid presents unique challenges due to the variable nature of sources like solar and wind. Smart grid-tie inverters equipped with adaptive control algorithms are emerging as a solution to these challenges. These advanced inverters can dynamically adjust their output based on grid conditions, enhancing stability and power quality.
Key features of smart grid-tie inverters include:
- Real-time monitoring and response to grid voltage and frequency fluctuations
- Active and reactive power control for grid support
- Fault ride-through capabilities to maintain grid connection during disturbances
- Communication interfaces for seamless integration with smart grid infrastructure
By employing these intelligent inverters, renewable energy systems can provide ancillary services to the grid, such as voltage regulation and frequency support, further increasing their value and integration potential.
High-frequency switching topologies for improved efficiency
High-frequency switching topologies are revolutionizing power conversion in renewable energy systems. By operating at frequencies well above traditional limits, these advanced converters can achieve higher power densities and efficiencies while reducing the size and weight of passive components.
The benefits of high-frequency switching include:
- Smaller magnetic components, leading to more compact designs
- Reduced switching losses, improving overall system efficiency
- Faster dynamic response to load and input variations
- Potential for higher power quality with reduced ripple and noise
As switching frequencies continue to increase, enabled by wide-bandgap semiconductors, the efficiency and power density of renewable energy conversion systems are expected to improve significantly.
Energy storage technologies enhancing conversion efficiency
Energy storage systems play a crucial role in maximizing the efficiency and reliability of renewable energy sources. By storing excess energy during peak production periods and releasing it when demand is high or production is low, these technologies help balance the intermittent nature of renewable sources like solar and wind.
Lithium-ion battery management systems for grid stabilization
Lithium-ion batteries have become the go-to technology for grid-scale energy storage due to their high energy density, long cycle life, and decreasing costs. Advanced battery management systems (BMS) are essential for optimizing the performance and longevity of these batteries in grid applications.
Modern BMS for lithium-ion batteries incorporate:
- Sophisticated state-of-charge and state-of-health estimation algorithms
- Thermal management systems to maintain optimal operating temperatures
- Predictive maintenance capabilities to prevent failures and extend battery life
- Fast response times for frequency regulation and load balancing
These features enable lithium-ion battery systems to provide critical grid stabilization services, enhancing the overall efficiency of renewable energy integration.
Flywheel energy storage in microgrid applications
Flywheel energy storage systems offer unique advantages for short-term energy storage and power quality improvement in microgrid applications. These mechanical batteries store energy in the form of rotational kinetic energy, providing rapid response times and high power output capabilities.
Key benefits of flywheel energy storage include:
- Millisecond response times for frequency regulation
- High cycle life with minimal degradation
- Environmentally friendly operation with no hazardous materials
- Ability to smooth out short-term power fluctuations from renewable sources
In microgrid environments, flywheels can work in tandem with other storage technologies to provide a comprehensive energy management solution, improving the overall efficiency and reliability of renewable energy systems.
Compressed air energy storage for load leveling
Compressed Air Energy Storage (CAES) systems offer a promising solution for large-scale, long-duration energy storage. These systems use excess electricity to compress air, which is stored in underground caverns or aboveground tanks. When energy is needed, the compressed air is released and heated, driving turbines to generate electricity.
CAES systems are particularly effective for load leveling applications, helping to balance supply and demand over longer time periods. They can store large amounts of energy for extended durations, making them ideal for integrating high penetrations of variable renewable energy sources into the grid.
Supercapacitors in hybrid energy systems
Supercapacitors, also known as ultracapacitors, are emerging as a complementary technology to batteries in hybrid energy storage systems. These devices offer extremely high power density and rapid charge/discharge capabilities, making them ideal for short-term power bridging and smoothing applications.
In renewable energy systems, supercapacitors can:
- Provide instantaneous power support during sudden load changes
- Extend battery life by handling high-power, short-duration events
- Improve overall system efficiency by reducing stress on other components
- Enable faster response times for grid frequency regulation
By combining supercapacitors with batteries and other storage technologies, hybrid energy systems can achieve superior performance and longevity, enhancing the overall efficiency of renewable energy conversion and utilization.
Machine learning optimization of energy conversion processes
The integration of machine learning (ML) and artificial intelligence (AI) into energy conversion systems is ushering in a new era of efficiency and performance optimization. These advanced algorithms can analyze vast amounts of data from sensors and operational metrics to make real-time adjustments and predict system behavior.
In solar energy systems, ML algorithms can optimize panel orientation and tracking systems to maximize energy capture throughout the day. By analyzing historical weather data and real-time conditions, these systems can anticipate cloud cover and adjust accordingly, minimizing losses due to shading and suboptimal angles.
For wind turbines, AI-powered control systems can optimize blade pitch and yaw angles based on complex wind patterns and turbine performance data. This dynamic optimization can increase energy output while reducing mechanical stress on the turbine components, leading to improved efficiency and longer operational lifespans.
Machine learning is not just enhancing individual components; it's revolutionizing entire energy conversion systems by enabling predictive maintenance, adaptive control, and intelligent grid integration.
In the realm of grid management, ML algorithms are being employed to predict renewable energy production and optimize energy distribution. These systems can balance supply and demand more effectively, reducing waste and improving overall grid efficiency. By analyzing consumption patterns and weather forecasts, AI can help utilities better integrate variable renewable sources and manage energy storage systems for maximum benefit.
Next-generation materials for photovoltaic energy conversion
The field of photovoltaic (PV) energy conversion is experiencing rapid advancements in materials science, pushing the boundaries of solar cell efficiency and durability. These innovative materials are set to revolutionize the solar energy industry, making PV systems more efficient, affordable, and versatile.
Perovskite solar cells: efficiency breakthroughs and stability challenges
Perovskite solar cells have emerged as one of the most promising technologies in photovoltaics, with efficiency rates rising at an unprecedented pace. These materials offer several advantages over traditional silicon-based cells:
- Higher theoretical efficiency limits
- Low-cost manufacturing potential
- Flexibility and lightweight properties
- Tunable bandgaps for optimized light absorption
However, perovskite cells face significant challenges in terms of long-term stability and durability. Researchers are working on innovative encapsulation techniques and material compositions to address these issues, aiming to bring perovskite solar cells to commercial viability.
Quantum dot solar concentrators for enhanced light absorption
Quantum dot solar concentrators represent an exciting development in PV technology. These nanocrystalline semiconductors can be tuned to absorb specific wavelengths of light, potentially allowing for more efficient harvesting of the solar spectrum.
Key advantages of quantum dot technology include:
- Improved light absorption across a broader spectrum
- Potential for multi-junction cells with higher efficiencies
- Reduced material costs through enhanced light concentration
- Compatibility with flexible and transparent substrates
As research progresses, quantum dot solar concentrators could lead to significant improvements in PV system efficiency and expand the applications for solar energy in building-integrated photovoltaics and other innovative designs.
Tandem solar cell architectures for exceeding Shockley-Queisser limit
Tandem solar cell architectures are pushing the boundaries of photovoltaic efficiency by combining multiple cell layers to capture a broader range of the solar spectrum. These multi-junction designs aim to exceed the Shockley-Queisser limit, which defines the maximum theoretical efficiency for single-junction solar cells.
Advanced tandem cell configurations include:
- Perovskite-silicon tandems for high-efficiency commercial applications
- III-V semiconductor tandems for space and concentrator photovoltaics
- All-perovskite tandems for low-cost, high-performance cells
- Organic-inorganic hybrid tandems for flexible and lightweight designs
By stacking cells with complementary absorption profiles, tandem architectures can achieve significantly higher efficiencies than single-junction cells, potentially revolutionizing the economics of solar energy production.
Waste heat recovery systems in industrial energy conversion
Industrial processes often generate significant amounts of waste heat, which represents a substantial opportunity for energy recovery and efficiency improvement. Advanced waste heat recovery systems are being developed to capture and utilize this thermal energy, reducing overall energy consumption and enhancing the sustainability of industrial operations.
Organic Rankine Cycle (ORC) systems have emerged as a versatile solution for converting low to medium-temperature waste heat into electricity. These systems use organic working fluids with lower boiling points than water, allowing for efficient power generation from heat sources that were previously considered unusable.
Thermoelectric generators (TEGs) offer another promising approach to waste heat recovery, particularly for small-scale and distributed applications. These solid-state devices convert temperature differentials directly into electricity, with no moving parts. While current TEG efficiencies are relatively low, ongoing research into advanced materials and nanostructured designs promises significant improvements in performance.
In addition to electricity generation, waste heat can be utilized for process heating, space heating, or absorption cooling systems. By integrating these heat recovery technologies into industrial energy conversion systems, companies can significantly reduce their energy costs and carbon footprint while improving overall process efficiency.
The implementation of waste heat recovery systems not only improves energy efficiency but also contributes to the circular economy concept by turning waste into a valuable resource. As industries strive to meet increasingly stringent environmental regulations and sustainability goals, these technologies will play a crucial role in optimizing energy use and reducing environmental impact.