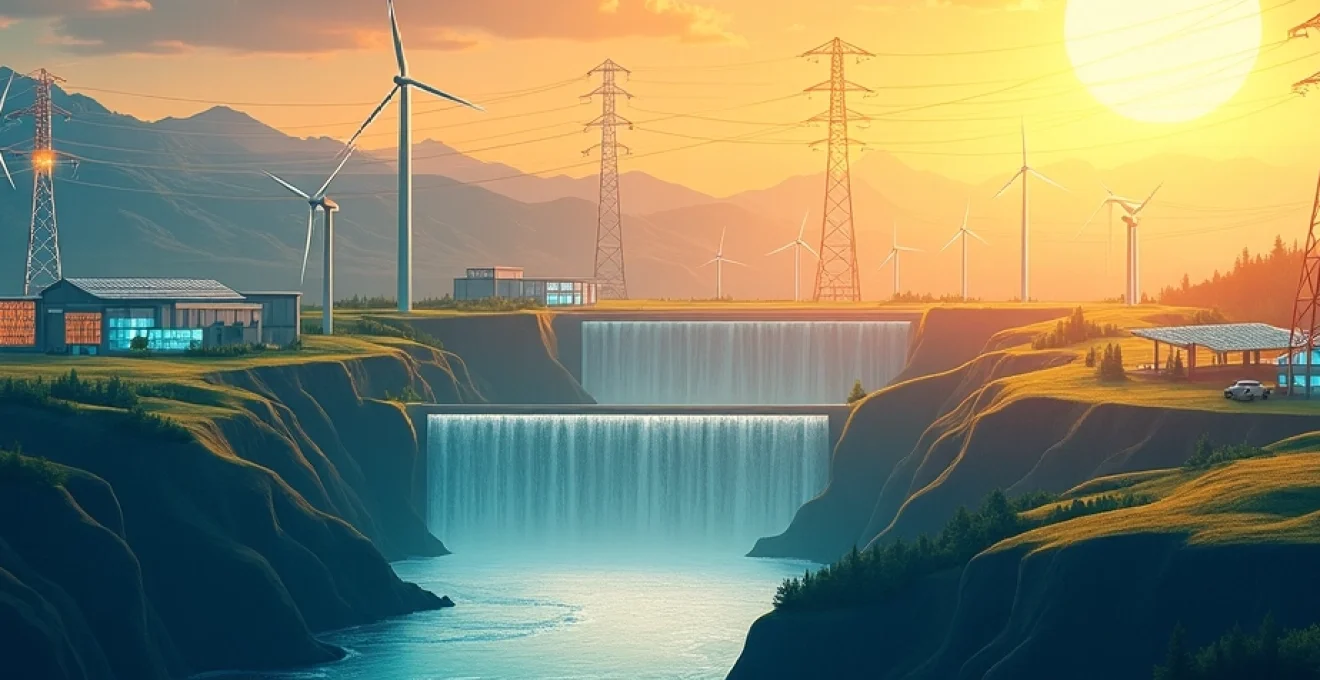
The transition to a sustainable energy future hinges on the successful integration of multiple renewable sources into a cohesive energy system. Hydropower, wind, and solar energy each offer unique advantages and challenges, but when combined, they create a robust and resilient power infrastructure. This synergy is crucial for meeting the growing global demand for clean, reliable electricity while mitigating the intermittency issues associated with individual renewable technologies.
As countries worldwide set ambitious targets for renewable energy adoption, the need for sophisticated integration strategies becomes increasingly apparent.
Fundamentals of multi-source renewable energy systems
Multi-source renewable energy systems represent a paradigm shift in power generation and distribution. These systems capitalize on the strengths of various renewable technologies to create a more balanced and efficient energy mix. The core principle lies in harnessing the complementary nature of different renewable sources to overcome individual limitations and enhance overall system reliability.
For instance, solar panels generate electricity during daylight hours, while wind turbines can operate day and night, provided there's sufficient wind. Hydropower, with its ability to store energy in reservoirs, can act as a buffer, releasing power when other sources are less productive. This interplay allows for a more consistent energy supply, reducing the need for backup fossil fuel generators.
The integration of multiple renewables also addresses the geographical and seasonal variations in resource availability. While solar irradiance may be lower in northern latitudes during winter, wind speeds often increase, compensating for the reduced solar output. Similarly, regions with abundant hydropower resources can supplement areas rich in wind or solar potential, creating a more balanced energy landscape across wider geographical areas.
Effective integration of diverse renewable sources is not just about combining technologies; it's about creating a symphony of energy production that responds dynamically to demand fluctuations and resource availability.
Advanced forecasting tools and smart grid technologies play a crucial role in orchestrating this complex interplay. By accurately predicting weather patterns and energy demand, system operators can optimize the contribution of each renewable source, ensuring grid stability and minimizing waste. This level of sophistication requires substantial investments in infrastructure and control systems, but the long-term benefits in terms of energy security and environmental impact are profound.
Hydropower integration techniques
Hydropower serves as a cornerstone in multi-source renewable energy systems, offering unparalleled flexibility and storage capabilities. Its integration with other renewable sources enhances overall grid stability and reliability. Let's explore several key techniques for optimizing hydropower's role in integrated renewable energy systems.
Pumped storage hydroelectricity for grid balancing
Pumped storage hydroelectricity (PSH) is a game-changer in managing the variability of wind and solar energy. This technique involves pumping water to an elevated reservoir during periods of excess electricity production, effectively storing energy for later use. When demand peaks or other renewable sources falter, the stored water is released through turbines to generate power.
PSH systems can respond rapidly to grid demands, providing essential load-following capabilities. They act as a giant battery for the grid, absorbing surplus energy during low-demand periods and supplying power during high-demand times. This flexibility is crucial for maintaining grid frequency and voltage stability in systems with high penetration of variable renewables.
Run-of-river systems in hybrid configurations
Run-of-river hydropower plants, which harness the natural flow of rivers with minimal water storage, can be effectively integrated with wind and solar installations. These systems provide a steady baseload power that complements the intermittent nature of other renewables. In hybrid configurations, run-of-river plants can operate continuously, filling the gaps when wind or solar generation is low.
The integration of run-of-river systems with other renewables often involves sophisticated control algorithms that optimize power output based on real-time data from all sources. This approach ensures maximum utilization of available resources while minimizing environmental impact, as these systems typically require less land inundation compared to large reservoir dams.
Small-scale hydropower in distributed networks
Small-scale hydropower installations play a vital role in distributed energy networks, particularly in remote or rural areas. These systems can be seamlessly integrated with local solar and wind resources to create resilient microgrids. By leveraging the natural topography and water resources of a region, small hydropower plants provide a reliable backbone for hybrid renewable systems.
The integration of small-scale hydropower often involves innovative designs that minimize environmental impact while maximizing energy output. For example, in-stream turbines can be deployed in existing water infrastructure, such as irrigation canals or water treatment facilities, adding renewable energy generation without significant additional construction.
Hydrokinetic turbines for continuous generation
Hydrokinetic turbines, which harness energy from flowing water without the need for dams or diversions, represent an emerging technology in hydropower integration. These turbines can be deployed in rivers, tidal streams, or ocean currents, providing a continuous source of renewable energy that complements the diurnal and seasonal patterns of wind and solar generation.
The integration of hydrokinetic turbines into multi-source renewable systems often involves careful site selection and environmental monitoring. Advanced materials and designs are employed to withstand harsh aquatic environments while minimizing impact on marine ecosystems. When combined with other renewables, hydrokinetic energy can significantly enhance the stability and reliability of coastal and riverine power grids.
Wind energy synchronization strategies
Wind energy plays a pivotal role in integrated renewable energy systems, offering significant potential for large-scale power generation. However, its variable nature necessitates sophisticated synchronization strategies to ensure seamless integration with other renewable sources and the broader power grid.
Offshore wind farms and grid connection challenges
Offshore wind farms present unique opportunities and challenges in the context of integrated renewable energy systems. These installations can harness stronger and more consistent winds compared to onshore facilities, potentially providing a more stable power output. However, connecting offshore wind farms to the onshore grid requires advanced transmission technologies and careful planning.
High-voltage direct current (HVDC) transmission systems are often employed to efficiently transport electricity from offshore wind farms to onshore substations. These systems minimize power losses over long distances and allow for better control of power flow. The integration of offshore wind with other renewables involves coordinating the timing of power delivery to match demand patterns and complement the generation profiles of solar and hydropower sources.
Vertical axis wind turbines in urban settings
Vertical axis wind turbines (VAWTs) offer a unique solution for integrating wind energy into urban environments. Unlike traditional horizontal axis turbines, VAWTs can operate effectively in turbulent wind conditions typical of cities. Their compact design allows for installation on rooftops or in small spaces, contributing to distributed energy generation in urban areas.
Integrating VAWTs with solar panels on buildings creates hybrid systems that can provide a more consistent energy supply throughout the day. Smart control systems can optimize the operation of these hybrid installations, balancing wind and solar generation to meet local energy demands and reduce strain on the central grid.
Wind forecasting models for optimal integration
Accurate wind forecasting is crucial for the effective integration of wind energy into multi-source renewable systems. Advanced forecasting models use machine learning algorithms and big data analytics to predict wind patterns with increasing precision. These models take into account factors such as topography, atmospheric conditions, and historical data to provide short-term and long-term forecasts.
By incorporating wind forecasts into grid management systems, operators can anticipate fluctuations in wind power generation and adjust other renewable sources accordingly. This predictive capability allows for more efficient scheduling of hydropower resources, optimal charging of energy storage systems, and better coordination with solar generation to ensure a stable and reliable power supply.
Power curve optimization in mixed energy systems
Power curve optimization is a critical strategy for maximizing the efficiency of wind turbines within integrated renewable energy systems. By fine-tuning the power output characteristics of wind turbines, operators can better match generation to grid requirements and improve overall system performance.
In mixed energy systems, power curve optimization involves adjusting turbine parameters based on real-time data from other renewable sources and grid conditions. For example, during periods of high solar generation, wind turbines might be operated at a slightly reduced capacity to prevent grid overloading. Conversely, when hydropower reserves are low, wind turbines could be pushed to their maximum efficiency point to compensate.
The art of integrating wind energy lies not just in capturing the wind, but in harmonizing its variable nature with the rhythms of other renewable sources and the demands of the grid.
Solar PV and thermal energy incorporation
Solar energy, both photovoltaic (PV) and thermal, is a cornerstone of integrated renewable energy systems. Its widespread availability and rapidly decreasing costs make it an attractive option for large-scale deployment. However, effectively incorporating solar energy requires innovative approaches to overcome its inherent variability and maximize its synergy with other renewable sources.
Concentrated solar power for baseload generation
Concentrated Solar Power (CSP) systems offer a unique advantage in integrated renewable energy systems by providing dispatchable power. Unlike traditional solar PV, CSP plants can store thermal energy in molten salt or other mediums, allowing for electricity generation even when the sun isn't shining. This capability makes CSP an excellent complement to variable sources like wind and solar PV.
In multi-source systems, CSP plants can be operated as baseload generators, providing a consistent power output that helps stabilize the grid. The thermal storage component allows CSP to shift its generation to evening hours, effectively filling the gap left by solar PV as the sun sets. This flexibility enhances the overall reliability of the renewable energy mix and reduces the need for fossil fuel-based backup generation.
Floating solar arrays on hydropower reservoirs
Floating solar arrays represent an innovative approach to integrating solar PV with existing hydropower infrastructure. By installing solar panels on the surface of hydropower reservoirs, these systems maximize land use efficiency and create a symbiotic relationship between solar and hydroelectric generation.
The benefits of this integration are multifaceted. The water body provides natural cooling for the solar panels, increasing their efficiency, while the solar arrays reduce water evaporation from the reservoir. Moreover, the two technologies can share existing power transmission infrastructure, reducing overall system costs. During periods of low solar irradiance, the hydropower component can compensate, ensuring a more stable power output.
Building-integrated photovoltaics in smart grids
Building-Integrated Photovoltaics (BIPV) represent a frontier in distributed solar energy generation. By incorporating solar cells into building materials such as windows, facades, and roofing, BIPV systems transform urban structures into power generators. This approach is particularly valuable in densely populated areas where traditional solar installations may be space-limited.
In the context of integrated renewable systems, BIPV contributes to the creation of smart, energy-efficient cities. When combined with smart grid technologies, BIPV systems can dynamically interact with other renewable sources and energy storage systems. For example, excess solar energy generated by buildings during peak hours can be stored or redirected to support local grid stability, reducing the strain on centralized power generation.
Solar thermal energy storage technologies
Solar thermal energy storage is a critical component in enhancing the reliability and dispatchability of solar power within integrated renewable systems. Unlike electrical battery storage, thermal storage systems can retain energy more cost-effectively over longer periods, making them ideal for smoothing out the daily and seasonal variations in solar energy availability.
Advanced thermal storage technologies, such as phase-change materials or thermochemical storage, are being developed to increase efficiency and reduce costs. These systems can be integrated with both CSP plants and distributed solar thermal collectors, providing a flexible energy reservoir that can be tapped when other renewable sources are underperforming or during peak demand periods.
Grid infrastructure and smart management systems
The successful integration of multiple renewable energy sources hinges on the development of robust grid infrastructure and intelligent management systems. These components form the backbone of a flexible, responsive, and efficient energy network capable of handling the complexities of diverse renewable inputs.
HVDC transmission for Long-Distance renewable integration
High-Voltage Direct Current (HVDC) transmission systems are pivotal in connecting remote renewable energy sources to urban centers and industrial hubs. HVDC lines offer significant advantages over traditional alternating current (AC) systems for long-distance power transmission, including lower losses and the ability to connect asynchronous grids.
In the context of multi-source renewable integration, HVDC technology enables the creation of energy superhighways that can transport large amounts of power from areas rich in renewable resources to regions of high demand. For example, HVDC lines can link offshore wind farms to onshore grids or transmit solar power from sun-rich deserts to distant cities, all with minimal losses.
Microgrid architectures for resilient power distribution
Microgrids play a crucial role in enhancing the resilience and flexibility of integrated renewable energy systems. These localized grids can operate independently or in conjunction with the main grid, providing a scalable solution for integrating diverse renewable sources at the community level.
In a multi-source renewable context, microgrids can dynamically balance local generation from solar PV, small wind turbines, and micro-hydro installations with energy storage systems and smart load management. This local balancing act reduces strain on the broader grid and enhances overall system stability. During grid outages, microgrids can island themselves, maintaining power supply to critical infrastructure and enhancing community resilience.
Demand response programs in Multi-Source systems
Demand Response (DR) programs are increasingly important in managing the variability of renewable energy sources. These programs incentivize consumers to adjust their electricity usage in response to grid conditions, effectively turning demand into a flexible resource that can be modulated to match renewable energy availability.
In integrated renewable systems, sophisticated DR platforms can coordinate with weather forecasts and real-time generation data from various sources. For instance, during periods of high wind generation, industrial processes might be ramped up to utilize the excess energy. Conversely, non-essential loads could be reduced during lulls in renewable generation, minimizing the need for backup power sources.
AI-driven predictive maintenance for hybrid plants
Artificial Intelligence (AI) and Machine Learning (ML) technologies are revolutionizing the maintenance and operation of hybrid renewable energy plants. Predictive maintenance systems use advanced algorithms to analyze data from sensors across different renewable installations, forecasting potential failures before they occur.
In multi-source systems, AI-driven maintenance strategies can optimize the performance of diverse technologies simultaneously. For example, an AI system might detect patterns indicating reduced efficiency in solar panels and schedule cleaning operations, while also adjusting the operational parameters of wind turbines to compensate for the temporary reduction in solar output. This holistic approach ensures maximum uptime and efficiency across the entire renewable energy portfolio.
Energy storage solutions for intermittency mitigation
Energy storage is the linchpin in creating stable and reliable multi-source renewable energy systems. As the penetration of variable renewable sources like wind and solar increases, the need for efficient and scalable storage solutions becomes paramount. These technologies not only mitigate the intermittency issues associated with renewables but also provide essential grid services such as frequency regulation and load balancing.
Advanced battery technologies, including lithium-ion, flow batteries, and emerging solid-state batteries, offer increasingly cost-effective solutions for short to medium-term energy storage. These systems can respond rapidly to fluctuations in renewable generation, smoothing out power delivery and maintaining grid stability. For longer-duration storage, pumped hydro remains a cornerstone technology, while innovative approaches like compressed air energy storage (CAES) and liquid air energy storage (LAES) are gaining traction.
Hydrogen energy storage represents another frontier in long-duration storage for integrated renewable systems. Excess electricity from wind or solar can be used to produce hydrogen through electrolysis, which can then be stored and later used in fuel cells or gas turbines to generate electricity when needed. This power-to-gas-to-power cycle offers a versatile means of seasonal energy storage, potentially solving one of the biggest challenges in 100% renewable energy systems.
Policy frameworks and market mechanisms for integrated renewables
The successful integration of multiple renewable sources into cohesive energy systems requires supportive policy frameworks and innovative market mechanisms. These structures must evolve to recognize and reward the value of flexibility, reliability, and sustainability that integrated renewable systems provide.
Feed-in tariffs and renewable portfolio standards have been instrumental in driving the initial growth of renewable energy. However, as the sector matures, new approaches are needed to address the complexities of integrated renewable systems. Capacity markets that value the ability to provide power during peak demand periods can incentivize investments in dispatchable renewables like CSP or biomass. Meanwhile, ancillary service markets that reward fast-responding assets can encourage the deployment of battery storage systems and smart grid technologies essential for balancing variable renewables.
Policy makers must also consider the broader system benefits of integrated renewables when designing support mechanisms. For instance, floating solar arrays on hydropower reservoirs provide not only clean energy but also reduce water evaporation - a critical consideration in water-stressed regions. Recognizing and monetizing such co-benefits can improve the overall economics of integrated projects.
Cross-border cooperation and regional grid integration play a crucial role in maximizing the potential of diverse renewable resources. Policies that facilitate power trading across jurisdictions can enable the development of complementary renewable assets, such as combining Scandinavian hydropower with North Sea offshore wind. These synergies can enhance system reliability and reduce the need for costly backup generation.
As we progress towards highly renewable energy systems, market designs must evolve to properly value flexibility. This includes implementing dynamic pricing structures that reflect real-time system conditions and creating markets for grid services like inertia and voltage support, which are traditionally provided by conventional generators but can be supplied by advanced renewables and storage technologies.
The transition to integrated renewable energy systems requires not just technological innovation, but also creative policy-making and market design to align economic incentives with system needs.
Successful integration of multiple renewable sources depends on a holistic approach that considers the entire energy system. Policies must encourage sector coupling - the integration of power, heat, and transport sectors - to unlock synergies and increase overall system efficiency. For example, using excess renewable electricity for heat pumps or hydrogen production can provide valuable flexibility while decarbonizing other sectors of the economy.
As we navigate this complex transition, ongoing research and international collaboration will be crucial. By sharing best practices and lessons learned, countries can accelerate the development of effective frameworks for integrated renewable energy systems, paving the way for a sustainable and resilient energy future.